Where are they heading next? Particles in the cleanroom
Cleanrooms and the protection of product carry two risks from contamination: particle deposition and particle dispersion. Airborne particle contamination in the healthcare and pharmaceutical sectors is a major topic of concern due to the risk of introducing particles, as impurities into products, and due to the potential to transfer microorganisms. Air quality assessments are partly based on the amount of particulate matter or particles in the air.
A cleanroom provides the environment necessary to manufacture and there are distinctive design elements intended to minimize particle ingress (HEPA filters and positive pressures); build-up (airflow); and retention (air changes). In order to further limit the contamination of particles, the source needs to be identified.
This article looks at the forces affecting the path particles might take within the cleanroom and whether or not a given cluster of particles are likely to settle.
The human factor
Multiple studies suggest humans to be the main cause for particle contamination in cleanrooms.¹ This contamination comes from skin cells and clothing fibers. Personnel can disperse fragments from their skin and particles from their indoor, non-cleanroom clothing.² With the skin losing over 20 million particles with a typical size of 30 µm per day this can be a significant factor to contamination.³
Suitable clothing is required in order to prevent the fibers coming off and the skin cells coming out of the suit. Face masks, gloves and hairnets are also necessary, albeit with some variation in clothing requirements depending on the application and products in the cleanroom.
The most significant microbial risk occurs when microorganisms settle out of the air and attach to a surface, under such conditions microorganisms, where sufficient nutrients and growth factors are available (including carbon, nitrogen and water), will thrive. This can not only lead to a contaminated surface, but it can also create re-contamination of the airstream since bacteria from the ground can be drawn into the airflow reaching other parts of the cleanroom, leading to a circle of contamination.
Yet not all cleanrooms are equal and the supply air filtration efficiency and the efficiency factor of ventilation system will considerably influence the concentration of particles in a given space.⁴
Particle deposition and particle dispersion
Particle deposition and dispersion are influenced by many factors including turbulence, indoor surfaces etc. The relative contributions are difficult to experimentally verify. Simulating and predicting the flow with the use of Computational Fluid Dynamics (CFD) can help with limiting particle dispersion and thus contamination of products.⁵
Particles in the air
Particles in the air are subject to different forces and hence they will behave in diverse ways, depending on their size. In the context of standard cleanroom monitoring, ≥0.5 µm particles are likely to behave differently to ≥5.0 µm particles.
Size and movement
The size of a particle causes it to move in separate ways. Three different phenomena are important ⁶,⁷:
- Brownian diffusion is characteristic of the random wiggling motion of small airborne particles. This is due to the constant bombardment by surrounding gas molecules. This phenomenon tends to make the concentration of particle within an area uniform. This is with particles typically less than ≤0.1 – 0.5 µm).
- For medium sized particles (such as ≥0.5 µm) these follow a variation of Stokes' Law, which enables an assessment of the settling velocity of sediment particles to be made. The settling velocity is dependent upon particle diameter, particle density, fluid density and viscosity of the carrier fluid.
In Stokes’s law, the drag force F acting upward in resistance to the fall is equal to 6πrηv, in which r is the radius of the sphere, η is the viscosity of the liquid, and v is the velocity of the fall.
This is idealized since a true interpretation of Stokes' Law requires particles to be truly spherical, smooth, and rigid in shape.
However, it is apparent that turbulent deposition and electrostatic attraction (Coulombic forces), which can influence all particle sizes, are more likely to affect particles within this range.
Gravitational settling is due to the gravity force exerted on a particle. This becomes the major motion force for particles with larger diameters (≥1 µm). The sedimentation effect is stronger than the buoyancy effect because the air density is much smaller than the particle density. Therefore, larger particles are more likely to settle.
Hence, particle diameter as well as the initial aerosol concentration affect the path particles are likely to take and can influence whether or not they might settle.⁸ This is one reason it is necessary not only to count particles but also to determine their size.
Particle deposition and particle dispersion
With reference to ‘turbulent deposition,’ this is influenced by a number of factors that can slow or increase air speed or lead to eddying and other changes to airflow. These become important cleanroom design factors to consider and can aid with risk assessment, especially in relation to any exposed product.
Particles and operational aspects
Operational aspects also affect the course particles might take. These are the general activities in cleanroom in terms of people and this is affected by the number of persons in the cleanroom and their activities, such as the use of tools.⁹
Other people related factors include the quality of the gown and the frequency and efficacy of disinfection.
Particles and temperature
Thermophoresis explains the movement of particles where the cleanroom air has temperature differences. This explains that when a temperature gradient exists on the enclosed volume: aerosol particles then tend to move from the high to the low temperature regions.
Particles and electrostatic effects
Electrostatic force can play a part when aerosol particles are charged and subject to an electrical field.¹⁰ Where the force is strong, it will counter most other forces.
Particles and surface proximity
The closer an air current containing particles is to a surface then the greater the possibility of deposition occurring. This has been shown by studies that generate particles towards a surface from different distances.
The more objects there are present in the air path (and the cleanroom overall) the greater the possibility of particle deposition. Preventing turbulence and fluctuations in the airflow is desirable since turbulence enhances particle dispersion, causing particles to not follow the airflow field. Furthermore, since particles cannot take the shortest path towards an extract, and have to go around equipment, this can cause airflow to slow, and this increases the possibility of deposition.¹¹
People, particles and proximity
Where there is a surface with objects on it and a person is required to be in close proximity (such as a person working at a desk within the cleanroom), particles are likely to deposit to a greater level on the surface (in this case a desk) since the airflow undergoes a further deviation from the operator’s head and this causes an increase in horizontal velocity.¹²
If this effect is applied to a critical area, such as the presence of product or components, such as an activity that breaks first air within a Grade A environment, then it can be seen that any detected particles are more likely to be directed downwards and in such cases practicing line clearance is a crucial step.
Particles and surfaces
Whether particles remain on a surface will vary according to surface materials and the orientation of the surface. Where microorganisms are present, time and the availability of nutrients will shape whether the organism becomes permanently bound to the surface.
Particle detection
In terms of detection, airborne particle counters provide a means to assess the totality of particles - either inert or microbial carrying¹³ although the relationship between these several types of particles is not easy to assess (an approximation can be made using biofluorescent counters). To assess airborne particles, particle counters use Mie scattering.¹⁴ This is based on the theory that describes the behavior of light scattering for particle in the size range of the light wavelength (in the case of cleanrooms, particle sizes are in the same order of magnitude as the wavelength of the light, which is in the range of 0.1 µm to 10 µm in diameter).
Larger particles have higher densities and are more likely to be detected.
Particle detection is limited by the metrology of the counter and its calibration (counters detect particles through the Mie scattering of particles moving through a light beam, and a proportion of the signal will reach the photodetector. It also follows that particles are not always truly diffuse within a given sample volume of air. The flow is often more even under laminar flow whereas they accumulate inside pockets within turbulent flow. Particles will also tend to rise in warmer regions. Hence, there will always be areas where particles congregate within the cleanroom.
Summary
This article has looked at the way particles of assorted sizes and concentration behave in the cleanroom.
In relation to conventional environmental monitoring, larger particles are more likely to be deposited onto surfaces (due to gravitational settling). This puts ≥5 µm particles as the size range that is most likely to settle. With ≥0.5 µm particles, while these are less likely to settle in an empty space, the presence of objects and the activities of people can affect the rate of turbulent deposition. Research by Whyte and colleagues places the division as settling to be influenced 80% by gravitational settling and 20% by turbulent deposition¹⁵, although this can alter through human activity.
Tim Sandle is a pharmaceutical microbiologist and writer.
References:
1. Vidali, S. (1992) Human contamination control in a clean room. Microchemical journal, 45(3):336–342
2. W Whyte and T Eaton. A cleanroom contamination control system. European Journal of Parenteral and Pharmaceutical Sciences, 7(2):55–61, 2002
3. Denina Hospodsky, Jing Qian, William W Nazaroff, Naomichi Yamamoto, Kyle Bibby, Hamid Rismani-Yazdi, and Jordan Peccia. Human occupancy as a source of indoor airborne bacteria. PloS one, 7(4):e34867, 2012
4. W. Sun, J. Liu, John M. K. Flyzik, et al. Development of Cleanroom Required Airflow Rate Model Based on Establishment of Theoretical Basis and Lab Validation. ASHRAE TRANSACTIONS, 2010, 116(1):87-97
5. Z Zhang and Q Chen. Prediction of particle deposition onto indoor surfaces by CFD with a modified lagrangian method. Atmospheric Environment, 43(2):319–328, 2009
6. W Whyte, M Derks, et al. Airborne particle deposition in cleanrooms: Relationship between deposition rate and airborne concentration. Clean Air and Containment Review, 25:4–10, 2016
7. Bin Zhao, Ying Zhang, Xianting Li, Xudong Yang, and Dongtao Huang. Comparison of indoor aerosol particle concentration and deposition in different ventilated rooms by numerical method. Building and Environment, 39(1):1–8, 2004
8. Whyte, W. , Agricola, K. and Derks, M. (2016) Airborne particle deposition in cleanrooms: relationship between deposition rate and airborne concentration. Clean Air and Containment Review, 25, pp. 4-1
9. Agricola, K., “Determination of operational quality of cleanroom by particle deposition monitoring,” Cleanzone 2013, (2013)
10. Jay R. Turner, Dimitris K. Liguras, H.J. Fissan, (1989) Clean room applications of particle deposition from stagnation flow: electrostatic effects, Journal of Aerosol Science, 20 (4): 403-417
11. Yun-Chun Tung, Shih-Cheng Hu, Tengfang Xu, and Ren-Huei Wang. Influence of ventilation arrangements on particle removal in industrial cleanrooms with various tool coverage. In Building Simulation, volume 3, pages 3–13. Springer, 2010
12. Sasan Sadrizadeh, Sture Holmberg, and Ann Tammelin. A numerical investigation of vertical and horizontal laminar airflow ventilation in an operating room. Building and Environment, 82:517–525, 2014
13. Sylvie Parat, Alain Perdrix, Sylvie Mann, and Pierre Baconnier. Contribution of particle counting in assessment of exposure to airborne microorganisms. Atmospheric Environment, 33(6):951–959, 1999
14. Hendrik Christoffel Hulst and Hendrik C van de Hulst. Light scattering by small particles. General publishing company Ltd, 1981
15. Whyte, W. , Agricola, K. and Derks, M. (2015) Airborne particle deposition in cleanrooms: deposition mechanisms. Clean Air and Containment Review, 24, pp. 4-9
Free Resources
Meet the author
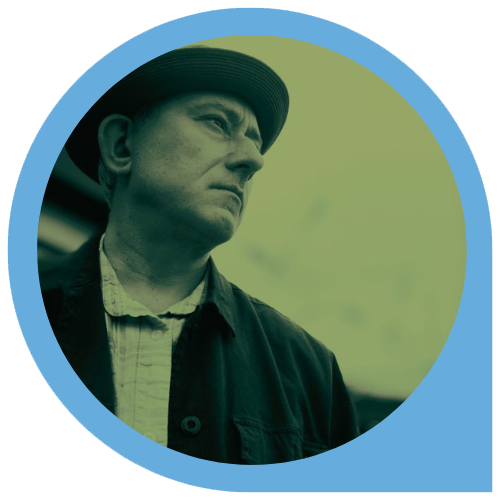
Pharmaceutical Microbiologist & Contamination Control Consultant and Expert. Author, journalist, lecturer, editor, and scientist
Tim Sandle, Ph.D., CBiol, FIScT
Tim Sandle is a pharmaceutical microbiologist and writer.